Tools of the astronomers: finding distance
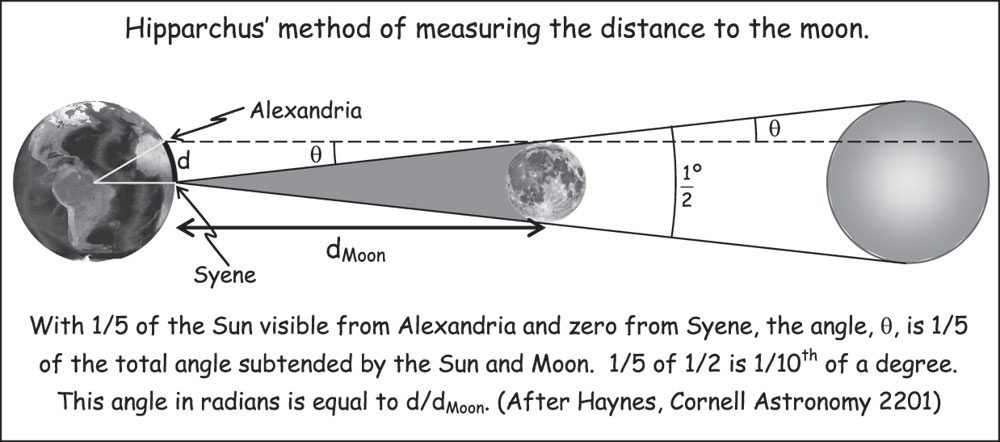
Through the years, I’ve written of the distances to stars and galaxies, but have never explained how astronomers have determined those distances. Finding these is actually one of the most challenging aspects of astronomers and measurements are still rife with uncertainty.
The first tool used to measure cosmic distances was parallax, the apparent change in position of an object due to the change in position of the observer. Most of us discovered parallax as bored children holding a pencil in front of our face, looking with one eye then the other and noticing that the pencil appeared to move. Most of us also realized that the distance the pencil appeared to move was smaller when it was held farther away from our eyes. In fact, there’s a simple trigonometric relationship between the angular shift in position (degrees of arc against the background), the separation of the eyes and the distance to the pencil.
The Greek astronomer Hipparchus measured the distance to the Moon in units of Earth’s radius (Aristotle argued the Earth was round based on the shape of the shadow it cast on the Moon during eclipses) during a total eclipse of the Sun. The eclipse was total in Syene, but in Alexandria it was only 80% of totality. As shown in the diagram, this gave the distance to the moon as the distance from Alexandria to Syene divided by 1/10th of a degree in radians. His measurements gave a distance was between 62 and 73 Earth radii (the uncertainty is because the distance units he used are not precisely known). We now know it to be 60.3 Earth radii away on average, so this method was very accurate. Thanks to the Apollo and other lunar missions, we have reflective cubes (corner cubes) on the Moon that reflect laser beams used to monitor its distance now.
Using careful observations and other geometry, the relative distances to the planets were known and used in the heliocentric model of the solar system presented by Copernicus so that once one distance was known accurately, the others were easily calculated. The advent of the telescope allowed for more accurate measurements of angles so Giovanni Cassini and John Flamsteed both measured the distance to Mars using parallax during its opposition in 1672. Opposition is when a planet is opposite the Sun in the sky. Cassini’s colleague, Jean Richer, traveled to Cayenne Island, off the coast of French Guiana to observe the opposition while Cassini remained in Paris. Flamsteed observed the position of Mars just after sunset one evening and before sunrise the next morning (at opposition, a planet rises at sunset and sets at sunrise), so that Earth’s orbital motion changed his viewing position (Earth’s average orbital speed is 66,000 mph). Both measurements resulted in the Earth-Sun distance being about 87 million miles. Our current measurement is 93 million miles on average. The method was excellent, but the accuracy was limited by their instruments.
Parallax is also used to measure the distances to stars. As Earth orbits the Sun, we see stars from opposite sides of our orbit. Aristotle argued against the heliocentric model of the Solar System because he did not see the star positions changing through the year due to parallax. An excellent argument, but incorrect due to the great distances to the stars. They do show parallax, but it’s tiny. Bright Sirius, a very close star at 8.6 light years, only shows a parallax of a mere 0.38 arcseconds, that is, 38 percent of 1/3600th of a degree. To measure this, we needed very precise instruments, indeed.
Unfortunately, with these tiny angles, we can only measure stellar parallax for stars closer than about 300 light years. Beyond that we need other methods.
Our primary tool for finding distances of distant stars and galaxies is comparing how bright they actually are to how bright they appear in our images since their brightness decreases as the square of the distance. The hard part is figuring out how bright they actually are! What we need are “standard candles”, objects whose intrinsic brightness can be determined from other characteristics.
Henrietta Leavitt, of the Harvard College Observatory discovered a very important class of standard candles in the first decade of the 20th century; Cepheid Variables. Named for Delta Cephei, an unremarkable appearing star in the constellation of Cepheus, these stars vary over regular periods. As she studied photographic plates of the Large and Small Magellanic clouds visible from the southern hemisphere, she found 47 Cepheid variables and noticed that their period of variation was proportional to their brightness. Thus, from measurements of the apparent brightness and the period of variation, a distance to these stars could be calculated. Edwin Hubble used Cepheid Variable stars in “spiral nebulae” such as the Andromeda Galaxy to determine their distances and conclude they were, indeed, “island universes”, not objects in our own galaxy. One of the major initial observing programs of the Hubble Space Telescope was to observe more Cepheid Variable stars in other galaxies to improve our measurements of their distances.
Another important standard candle is the brightness of Type Ia supernovae. These explosions occur in binary star systems where one star has evolved through red giant and planetary nebula stages into a white dwarf star while the other is still in the red giant stage. The white dwarf star is actually able to pull material off the bloated red giant so its mass continually increases. When that mass goes beyond 1.4 times the mass of the Sun, the white dwarf explodes as a Type Ia supernova. Since these explosions all have the same origin, they should attain the same peak brightness. Fortunately, they have a unique spectral evolution different from other supernovae so they can be identified. The hard part is identifying them before they reach their peak brightness (which occurs within days of the initial explosion). This has led to a cottage industry of supernova hunters attempting to find these transient phenomena in distant galaxies. In the early part of this century the fruits of these efforts led astronomers to the startling conclusion that the expansion rate of the universe, initially determined by Edwin Hubble with the aid of Cepheid Variable stars, is increasing.
Without the aid of a Cepheid Variable or Type Ia supernova, it’s quite a the challenge to find distances to other galaxies. In my next column, I’ll discuss some of those.
In the meantime, the volunteer astronomers at the Adirondack Public Observatory are eager to point out all the stars of Orion and offer views of the cosmos through our telescopes. The Roll Off Roof Observatory (RORO) is open to the public on the first and third Fridays of each month approximately one half-hour after sunset. Whether you’re an avid amateur astronomer or have never visited an observatory, come and view through our telescopes and learn about the Wilderness Above. For updates and notices, check out our website at adirondackpublicobservatory.org and our Facebook page. On our public observing days you can also call the RORO at 518-359-6317 to talk with one of our astronomers.